The successful sequencing of the human genome in 2003 has opened the door to a broad range of scientific and commercial opportunities and challenges.
The expanding ability to acquire health information from genetic tests and apply it to screening, prevention, diagnostics, and therapeutics has already fostered enormous changes in clinical medicine, not the least of which is the ushering in of the precision medicine era.
As the pace of investigation and discovery in this area continues to expand and accelerate, genetic testing is now playing an increasingly pivotal role in clinical medicine as well as in life and health insurance.
Information from genetic tests has the potential to improve population mortality and morbidity experience, but challenges still exist for insurers in using such information. Many countries continue to prohibit genetic test results for use in underwriting, even if the results have been shared with an insurer. In addition, as more individuals order their own genetic tests, a growing asymmetry of information is potentially emerging. This may create added challenges and could signal a significant shift in the information balance between consumer and insurer.
The impact of genetic testing on the insurance industry will continue to be an important topic of actuarial research for some time as insurers address its effect on pricing and valuation. Insurance product development opportunities may also emerge to capitalize upon the genetics revolution that has the potential to benefit both the consumer and the insurance industry.
The ultimate objective would be for insurers to partner with insureds so as to leverage genetic advances for mutual benefit and to promote longevity and improve quality of life. RGA is strongly optimistic about the potential for genomic medicine to drive morbidity and mortality improvements and increase healthy life expectancy.
This paper is a revision of a review published by RGA in November 2016. Since that time, the field of genomics has progressed so rapidly that a significant update was needed.
Genetics and Genetic Testing
Human genetic information is contained within the 23 chromosomes found in nearly every cell of the human body The chromosomes contain DNA, which is arranged in a very specific linear sequence of three billion chemical base pairs that are organized in a double helix configuration. Within those base pairs are the approximately 23,000 protein-coding genes, also known as the exome. However, these protein-coding genes make up only 2% of total human DNA. The remaining 98% of the noncoding DNA regulates gene expression and may serve other, as yet unknown, functions. See Figure 1 (below).
Figure 1:
The Genetic Code
Source: Cancer Research UK / Wikimedia Commons1
The sum total of all DNA, both coding and non-coding genes, is called the genome.
Genetics is a term that refers to the study of genes and their roles in inheritance. Genomics is a more recent term which describes the broader study of the genome, including interactions of genes with one another and with a person’s environment.2
Genomics and genetic tests analyze how genes contribute to health and disease and are playing a greater role in the development of gene-based treatments. Not all genetic tests are the same Inexpensive genome-wide association studies (GWAS), for one, use microarray (gene chip) technology to examine genome-wide sets of genetic variants and produce genotypes. The single base pair genetic variants known as single nucleotide polymorphisms (SNPs), for example, may be associated with factors such as a higher or lower predisposition to develop a disease or a propensity to experience side effects from certain drugs (See Figure 2 ). From these studies, polygenic risk scores, which measure predisposition for certain genetic conditions, can be calculated.
Figure 2:
Single nucleotide polymorphism (SNP). Example demonstrates a C to T variant.
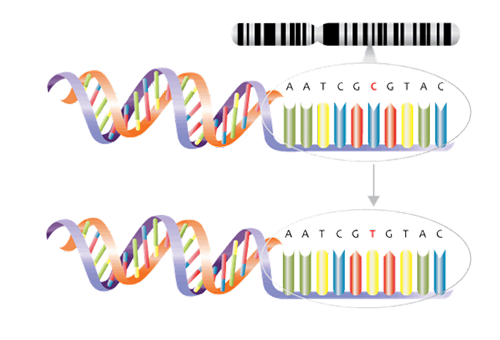
Source: NHS National Genetics and Genomics Education Centre1a
Other genetic tests include whole genome sequencing (WGS), which decodes every single base pair, and whole exome sequencing (WES), which only decodes the protein-coding regions. Targeted gene sequencing panels, another type of test, consist of laboratory panels assembled to contain a preselected or custom-designed set of genes or gene regions with known or suspected associations with particular diseases.3 These can be used if an individual has symptoms of illnesses with genetic components such as cancer, or a family history of such illnesses. These panels enable searches within a genome for specific variants associated with the impairments in question without the need to sequence the entire genome or exome, and can assist with diagnosis, prognostication, and treatment decisions.
The Science, Validity, and Utility
Genetic tests, like any other medical tests, can yield false positive as well as false negative results. Whether a genetic test is applicable in a given clinical situation depends on three factors: the test’s analytical validity, clinical validity, and clinical utility, as defined in Table 1.
Table 1: Genetic Test Criteria |
Analytical Validity | The ability of a genetic test to detect and measure the presence of a genetic variant of interest accurately and reproducibly |
Clinical Validity | The ability of a genetic test to divide a population into two or more groups on the basis of risk or outcomes |
Clinical Utility | The ability of a genetic test to demonstrate improvement in the outcomes (prevention, prognosis, diagnosis, treatment, or management) of an impairment. Often the term “actionable” is used when considering clinical utility. |
Source: Adapted from Merker JD, et al. J Clin Oncol.4
In terms of analytical and clinical validity, genetic tests can reliably find variants, but as gene panels do not test for all types of genetic variants, the possibility of false negative results exists. Also, simply having a genetic variant does not necessarily translate into clinical manifestation. This aspect, termed penetrance, is defined as the likelihood of a disease developing when the variant is present.
Clinical utility refers to whether a doctor who gains the knowledge that a patient has a specific genetic variation might change how that doctor medically manages that patient. This can translate to whether a physician might motivate patients with potentially disease-causing mutations to change behaviors or institute preventative strategies that might materially improve clinical outcomes. For example, a 2013 study found that approximately 3% of adults carry high-penetrance, actionable, and pathogenic or likely pathogenic genetic variants. Knowledge of these variants could enable doctors to tailor patient care to prevent disease expression.5 However, many critics of genetic testing argue that the presence of a genetic variant might not yet be a strong enough argument for using genetic information in clinical care unless there is a substantial adverse family history or clinical signs and symptoms of a particular disease.
Variants
Genetic research has determined that all humans, no matter their geographic origin, are about 99.5% the same genetically.6 Studies of twins have shown that approximately 25% to 50% of morbidity and mortality differences from person to person are due to genetic variation.7,8 The remaining variation in disease rates is due to environmental, behavioral, and lifestyle factors.
Two recent studies clearly demonstrate the impact of non-genetic factors on outcomes, independent of genetic determinants. One, published in 2016, looked at the genetic component of cardiovascular (CV) disease risk, based on polygenic risk scores (PRSs) (see page 8). It found that individuals with favorable lifestyle factors had a 46% lower relative CV risk than those with unfavorable lifestyle factors, despite the equally high genetic risk.9 A more recent study, conducted in 2018 and based on UK Biobank data, demonstrated that an unfavorable lifestyle profile was associated with an increased risk of stroke across all genetic risk strata. The findings highlight the potential of lifestyle interventions to reduce the risk of stroke across entire populations, even for those at high genetic risk for stroke.10 While genetics has an influence on outcomes, behavior, and lifestyle also have a significant impact and can mitigate genetic risk factors.
A typical individual’s exome sequence may have approximately 40,000 variants, and a whole genome sequence approximately three million. Variants in human DNA, which are often referred to as mutations, can lead either to disease, or to protection from disease, or might have little to no impact at all.
Table 2 describes the types of genetic variants which have been discovered and the likelihood that they might be pathogenic.11 The vast majority of genetic variants currently fall into the category of “variant of unknown significance” (VUS). Additionally, variants can be described as likely benign or benign. Over time, as more is learned about these VUSs, many will likely be reclassified.
Table 2: Types of Genetic Variants |
Term | Probability Variant is Pathogenic |
Pathogenic | > 99% |
Likely pathogenic | 90-99% |
Variant of unknown significance (VUS) | < 90% |
Source: Biesecker LG, et al. Distinguishing Variant Pathogenicity from Genetic Diagnosis. JAMA 2018.
While genetics has influence on outcomes, behavior and lifestyle also have significant impact and can mitigate genetic risk factors.
Market Factors
Public access to genetic testing has been increasing rapidly. Today it is available through clinicians, employee health programs, and direct-to- consumer (DTC) genetic testing kits.
The number of types of clinical genetic tests available has also increased markedly as DNA sequencing costs have plummeted. Approximately 75,000 genetic tests are currently on the market, and on average 10 more are added each day. According to a genetic testing claims database, prenatal genetic tests accounted for 33% to 43% of spending on genetic tests and hereditary cancer tests for about 30%.12
The cost of these tests has dropped precipitously as well. Today they can range from less than US$100 to US$4,500, depending on testing methodology and completeness of the assay The cost of whole genome sequencing, for example, has decreased significantly in the last 15 years and is now approximately US$1,000.
The global genomics market size is expected to reach US$27.6 billion by 2025, progressing at a compound annual growth rate of 9.7%.17
Figure 3:
DTC Genetic Tests Performed by Year

Source: Adapted from Regalado A. MIT Technology Review.
The global DTC testing market has also been experiencing substantial growth. In 2017 it stood at US$99 million, more than double that of the prior year according to an article in MIT Technology Review. By 2022 it is expected to grow to US$322 million, and by 2024 is expected to surpass US$2.5 billion.13 Most DTC tests were purchased in the U.S., which would suggest that approximately 4% of American adults now have access to some form of their personal genetic data.14 The growth of DTC testing has been significant, with more than 14 million tests performed in 2018 alone. Indeed, by the beginning of 2019 more than 26 million people had contributed their DNA to the four leading commercial ancestry and health databases.15
DTC testing, however, is not without controversy. For example, on March 6, 2018, the U.S. Food and Drug Administration (FDA) granted the first marketing authorization for DTC tests by 23andMe of three BRCA1 or BRCA2 mutations. Permission was granted based on the accuracy and reproducibility of the test (that is, its analytical validity). However, in its permission, the FDA noted that “most BRCA mutations that increase an individual’s [breast cancer] risk are not detected by this test."16
Therefore, concern is rising that a false sense of security might be conferred by a negative test. Some countries, such as France and Germany, require all genetic tests – including DTC tests – to be ordered by a physician, who can then explain and interpret the test results for patients.
New Tests
Polygenic Risk Scores
Geneticists have long observed that certain SNPs and SNP patterns occur more frequently in people with a particular disease or complex trait. As GWAS sample sizes have increased, more SNPs associated with disease have been discovered, which can then be used to predict an individual’s risk of the morbidity. Risk can be assessed using a polygenic risk score (PRS), a metric that condenses information from tens, hundreds, thousands or even millions (“poly”) of a person’s genetic variants (“genic”) into a score that measures the individual’s genetic predisposition to a disorder.
These scores are often expressed as risk percentiles. An individual with a PRS in the 99th percentile for diabetes, for example, would be considered to have a high genetic risk. However, for an individual with a PRS closer to the population mean (50th percentile), the score would offer no additional risk information: the person’s predicted risk, based on his or her genetics, will be similar to the population’s average risk.
Researchers have now developed PRSs for many common diseases and have shown their potential in risk prediction. For example, a PRS for coronary artery disease risk has demonstrated that people with a PRS in the highest 5% have a threefold increased risk of experiencing the condition compared to individuals with lower PRSs.9 In a study on genetic risk and breast cancer, women whose PRSs were in the top 20% were shown to have a 17.2% lifetime risk of breast cancer compared to a 5.3% lifetime risk for women whose scores were in the lowest quintile.18
RGA has been collaborating with King’s College London since 2016 on research utilizing UK Biobank data. The research is aimed at gaining a better understanding of the value of polygenic risk scores to predict disease and death. A key focus of it is to examine whether PRSs provide additional risk information not captured by routinely collected clinical and biomarker data.
Initial results demonstrate that PRSs could make a significant contribution to risk prediction for incidence of death from breast cancer and coronary artery disease, above and beyond typical underwriting risk factors. This raises the possibility of adverse selection, where genetic information about common disorders is available to insurance purchasers but not underwriters. These results have been presented at numerous actuarial conferences around the world and are discussed in detail in a series of RGA webcasts. To access, please follow this link: https://www.rgare.com/knowledge-center/media/videos.
Further research is imperative to understand how PRSs and other advances in genomic medicine could cause adverse selection if consumers use this information to alter their insurance purchasing behavior. Nonetheless, the hugely positive impact genomic medicine is likely to have on reducing morbidity and mortality will help to mitigate this risk.
Epigenetic Testing
Epigenetics is an emerging field of science that studies heritable changes that cause the activation and deactivation of genes without any changes in the underlying DNA sequence. The word “epigenetics,” of Greek origin, literally means “over and above the genome”19 and refers to how over time environments (e g , exposure to pollutants), experiences, and lifestyle choices (e.g., diet, nutrition, toxins) can induce processes that change how human genetic codes are expressed. The changes are characterized by DNA methylation, histone modifications, or higher-order structural modulation of chromatin (the components of chromosomes). While heritable, many epigenetic changes can be reversed.
It is believed that the study of epigenetics or of the epigenome, as it is now called, may be able to predict all-cause mortality risk20 as well as qualify and quantify the use of tobacco and consumption of alcohol. Many factors emerging as having potential epigenetic influence are not captured in either the traditional medical record or underwriting processes. Thus, epigenetics may have the potential to offer truly novel information.
Because epigenetic testing does not refer to the actual DNA sequence, some might argue it should not be subject to many of the current regulatory restrictions surrounding genetics. However, this question has not been specifically addressed, and until it is it will be difficult to predict how it might be viewed in the future.
Genetic Testing and Cancer
Cancer is a genetic disease and genetic testing has revolutionized the field of oncology. Testing can now be performed on germline as well as tumor cells to identify the variants and mutations which may drive certain cancers. This has enabled the discovery of new targeted biological anti-cancer therapies, many of which have been shown to improve cancer survival in the approximately 10% to 15% of cancer patients with advanced disease. A 2016 study reported that 91% of cancer patients had actionable mutations (that is, mutations with significant diagnostic, prognostic, or therapeutic implications for cancer patients and their families), and that 10% of treatment plans were altered as a result of genetic testing of their cancer genome done at the time of the cancer diagnosis.21 Unfortunately, these novel targeted cancer medications are very expensive right now and, in many cases, only extend life expectancy marginally.
An April 2018 study examined the percentage of U.S. patients with advanced or metastatic cancer who benefited from genome-driven oncology between 2006 and 2018. Utilization of genome-informed cancer therapy rose from 10.5% to 15.4% over this time period. Those actually benefiting from this therapy increased from 1.3% in 2006 to 6.6% in 2018. The median overall response rate for all genome-informed drugs through January 2018 was 54%, with a median duration of response of 29.5 months. The authors concluded that these novel drugs have helped only a minority of patients with advanced cancer.22
For further reading please see an article by Dr. John Lefebre in the May 2018 issue of ReFlections, RGA’s global medical newsletter. To access, please click on this link https://www rgare com/knowledge-center/article/understanding-the-genetics-of-cancer.
Liquid Biopsies
Considerable focus and research has been directed in recent years toward the development of liquid biopsies. These tests, which look for biological and genetic markers of cancers in blood or other bodily fluids, have been studied for screening and therapeutic purposes, including the surveillance of recurrent or metastatic disease. There is a high expectation that these tests may have a significant and beneficial impact on the mortality and morbidity outcomes of cancer in the coming years. There is also some concern these tests could impact cancer incidence rates and cancer diagnostic definitions and thus challenge actuarial pricing assumptions for living benefits products.
For a white paper providing a detailed review of this testing and RGA’s opinion, please click on this link: https://www rgare com/knowledge-center/article/liquid-biopsy-the-rga-perspective.
Pharmacogenomics (PGx)
The growing field of pharmacogenomics, often abbreviated PGx, is the study of how genes affect a person’s response to drugs. Pharmacogenomics is increasingly enabling the provision of the right drug at the right dose at the right time in order to achieve better efficacy and reduce potential side effects. More than 250 FDA-approved drugs are now labeled for prescribing based on the patient’s genes – a number which has tripled since 2014.23 Also, in October 2018, the FDA authorized the first DTC test, the 23andMe Personal Genome Service Pharmacogenetic Reports test, which can detect 33 genetic variants that could be associated with variations in medication metabolism.24
As serious adverse reaction to medications is common in clinical medicine, increased integration of pharmacogenomics into clinical medicine could enable greater precision in prescribing and treatment, leading to significant decreases in drug side effects, improved disease outcomes, and ultimately, improved morbidity and mortality. Thus, pharmacogenomics is a cornerstone of the growing field of precision medicine.
Other Innovations in Genetics
Gene Editing
Scientists have been searching for ways to edit genes since the 1960s. However, the discovery of CRISPR-Cas9 (clustered regularly interspaced short palindromic repeats/ CRISPR-associated protein 9) technology has brought science closer to this capability.
Gene editing is a group of technologies that is enabling scientists to modify an organism’s DNA – including that of humans. These technologies allow genetic material to be added, removed, or altered at particular locations in the genome CRISPR-Cas9, which is regarded by many scientists as a “molecular scalpel,” has generated substantial excitement because it is faster, cheaper, more accurate, and more efficient than other gene editing methods. This innovative technology is of great interest in the prevention and treatment of human diseases, but most current research is being conducted using cellular and animal models. Researchers are still working to determine whether this approach is safe and effective for use in humans.25
Even though most CRISPR-Cas9 research is focused on single-gene disorders such as cystic fibrosis, hemophilia, and sickle cell disease, this technology also holds out hope for the treatment and prevention of more complex diseases such as cancer, heart disease, mental illness, and human immunodeficiency virus (HIV) infection.
Gene editing technology also brings up a number of ethical challenges, as these tools have the capacity to alter human genomes. Even though most of the changes introduced with gene editing are limited to somatic cells, gene manipulation could be used to modify germline cells (egg or sperm cells) and be heritable. Based on issues about ethics and safety, germline cell and embryo gene editing are currently illegal in many countries.26
Despite multiple unknowns, a scientist in China announced in November 2018 the use of the CRISPR-Cas9 gene-editing tool to modify the CCR5 gene, which confers resistance to HIV, in two human embryos, which were then implanted and brought to term. According to published reports, this revelation was met with widespread criticism. The International Human Genome Editing Summit, where the doctor presented his data, rebuked him and said his claim was deeply disturbing and irresponsible. Since then, the World Health Organization has announced plans to establish a panel to develop gene-editing standards.27
The next few years will show us whether this revolutionary technology can ever be translated into safe and ethical human clinical trials.
Gene Therapy
In this process, one or more genes are inserted into a person’s cells to correct for the presence of an inherited disease-causing genetic mutation. The resulting new, normal-functioning gene is referred to as a transgene. The limiting factor with this type of therapy to date is finding an efficient way of delivering (e g , by a vector) the constructs for gene therapy to a person’s cells. The majority of vectors are derived from viruses, with the adenovirus vector appearing to be the most efficient delivery system to date.
Gene therapy is unique in medicine as the goal is to treat the cause of a particular disease, not its symptoms Its use in clinical therapy is limited at this point in time, but it still holds great promise to revolutionize the management of human diseases.27 Sixteen of the 46 new molecular entities as well as three gene therapies approved by the FDA in 2017 are considered “personalized” medicines, which means prior use testing is performed to determine if those therapies will work best for individual patients.28
Conditions that appear treatable via gene therapy include retinal defects,29 hemophilia, cystic fibrosis (a single-gene disorder), and certain hemoglobinopathies (inherited single-gene disorders) such as sickle cell disease and thalassemia. One current example of gene therapy is the use of voretigene neparvovecrzyl to treat Leber’s congenital amaurosis 2 (LCA2), a specific form of retinitis pigmentosa, in individuals with confirmed biallelic RPE65 mutations. In a Phase 3 randomized controlled trial of 31 patients aged ≥3 years with LCA2, bilateral subretinal delivery of voretigene neparvovec (AAV2 vector) improved their ability to navigate independently in low-to-moderate light conditions at one-year follow-up, compared with controls. In addition, nearly all treated patients showed improved light sensitivity, visual fields, and functional vision under dim lighting conditions.30 This therapy is the first gene therapy approved by the FDA (December 2017) for an inherited disorder. The cost of a one-time treatment is approximately US$850,000. To date, no serious adverse effects have been reported, but it remains to be seen if the results will be durable.
Another example of gene therapy is chimeric antigen receptor-T (CAR-T) or T-cell immunotherapy, which is used to treat certain lymphoid malignancies. In this therapy, a patient’s own T lymphocytes are extracted from their blood and a gene for the chimeric antigen receptor (CAR) is injected into them, creating CAR-T cells. Millions of these cells are then grown in the lab and subsequently infused back into the patient, where they bind to a specific antigen on the cancer cells, killing them.
Tisagenlecleucel was the first CD19 (a B-cell surface protein) antigen-specific CAR-T cell therapy approved by the FDA (2017) for patients 25 years of age or younger with relapsed or refractory B-cell precursor acute lymphoblastic leukemia (ALL). One recent Phase 1 study of adults with relapsed B-cell ALL showed that the overall median survival with this form of treatment was 12.9 months.31 The one-time infusion cost of US$475,000 of tisagenlecleucel makes it the most expensive oncologic therapy today.
Axicabtagene ciloleucel is another example of CAR-T immunotherapy. It was approved by the FDA in 2017 for treatment of adults with relapsed or refractory diffuse large B-cell lymphoma (DLBCL) after two or more lines of systemic therapy. One Phase 2 study enrolling patients with refractory lymphoma after undergoing conventional therapy showed an overall survival rate at 18 months after treatment of 52%.32 At this point, however, the U.K. ’s National Institute for Health and Care Excellence (NICE) has recommended against the use of axicabtagene ciloleucel. In a draft guidance dated August 29, 2018, NICE has said it is not clear how much of a benefit axicabtagene ciloleucel may provide compared with salvage chemotherapy. Additionally, the cost of a one-time infusion of axicabtagene ciloleucel, at US$373,000, is too high for it to be considered a cost-effective use of National Health Service (NHS) resources.
As can be seen, the cost of these therapies can be substantial and it has yet to be shown that their use is cost-effective as salvage therapy or relative to traditional therapies.
Undoubtedly the clinical armamentarium of gene therapies will grow in the future, with high expectations of reducing morbidity and mortality. However, costs, patient access, and resource allocations may present significant barriers.
Risks and Benefits for Insurers
Genetic testing is an emotionally charged and controversial topic for the public, for lawmakers and regulators, and for the insurance industry. There has also been frequent commentary on the public’s concern that the use of their genetic information might impact their insurability, which could reduce their willingness to participate in genetic research studies – something insurers should bear in mind. Thus, for insurers, although there are possible benefits in genetic testings’ use, there are also several possible risks.
Regulations and Anti-Selection
Currently, no insurance market anywhere in the world requires genetic tests to be ordered at the time of life or health insurance underwriting. Laws vary by country and product as to whether clinically obtained and disclosed genetic tests results can be used during underwriting, and these laws are constantly evolving. Most countries currently follow the general principle that applicants must declare what they know about all aspects of their health in order to keep the insurance contract equitable.
The U.K. as well as some European Union members have agreed upon insurer moratoriums on the use of genetic information and require underwriters to ignore any genetic test results – even if disclosed – if the sum assured being applied for is below a certain threshold. In the U.K., for example, the use of Huntington’s disease genetic information is allowed when underwriting life policies with face values of greater than £500,000. The Association of British Insurers (ABI) and Government updated its Code on Genetic Testing and Insurance (previously called the Concordat and Moratorium on Genetics and Insurance) in October 2018. This update is the sixth iteration of a long-standing agreement. While the name has changed, the overall directive has not: the only real change is that the agreement now has no expiration date and can be reviewed at any time.33
Other countries have imposed a complete ban on underwriting cases based on the results of any disclosed genetic test results. In 2017, Canada enacted the Canadian Genetic Non-Discrimination Act, which prohibits any person from requiring an individual to undergo a genetic test or disclose the results of a genetic test as a condition of providing goods or services to, entering into or continuing a contract or agreement with, or offering specific conditions in a contract or agreement with, the individual. Exceptions are provided for health care practitioners and researchers. The law clearly applies to insurers and as a result, adjustments to underwriting practices in Canada have been implemented.34 Legal challenges to this law have now begun in Quebec.
In the U.S., the Genetic Information Nondiscrimination Act of 2008 (GINA) is a federal law that prohibits genetic discrimination in health insurance and employment but does not apply to life, disability, and long-term care cover. Additionally, there are some other underwriting restrictions in individual U S states depending on insurance product.
In Australia, the Financial Services Council recently completed a consultation draft of its Life Insurance Code of Practice, which includes a moratorium on genetic tests in life insurance, and solicited feedback through the end of January 2019. The moratorium will now become an FSC Standard and insurers may only ask for or use the results of a previously obtained genetic test if the aggregate cover is greater than specified amounts based on product type. Insurers must also take into account any favorable genetic information an applicant chooses to disclose. The moratorium is set to go into effect July 1, 2019 and will be effective through June 30, 2024, with a review in
2022. 35,36
As for anti-selection, a study conducted more than 10 years ago demonstrated that there is a 5.7-fold increased tendency for policyowners to change their long-term care insurance coverage if they know they carry genes associated with increased risk for Alzheimer’s disease.37
RGA has been a consistent advocate for a level, free, and fair exchange of information between the insurer and the consumer. This can provide a good opportunity for partnership going forward in terms of leveraging the information for shared benefit and ultimately lower prices for consumers.
Impact on Product Pricing
The definitive impact on product pricing due to information asymmetry that might stem from insurers’ ability (or lack thereof) to access genetic testing information is currently unknown. Genetic testing may impact both mortality and morbidity outcomes and influence in-force lapse rates. For example, someone receiving favorable genetic test results may be more reassured and allow a policy to lapse, while someone receiving concerning results may be more motivated to keep their policy in force, thus negatively impacting the overall mortality expectations of the remaining pool. Much uncertainty exists, and assumptions are needed in two categories: impact on consumer behavior, and impact on mortality and morbidity outcomes.
Research conducted in 2011 found that the effect of restricting the use of genetic test results in underwriting would be about a 1% to 3% increase in premiums. However, only six genetic disorders were included in that analysis.38 A more recent report (2014) from the Canadian Institute of Actuaries, which examined 13 impairments with a known genetic marker, found that banning the use of genetic test results in underwriting could increase average mortality rates by 35% for males and 60% for females.39 The same author, in a 2016 report, demonstrated that a ban on using genetic information in critical illness underwriting would result in a 26% increase in the average CI claims rate (+16% for males and +41% for females),40 and could necessitate an increase in CI premium rates.
The Institute of Actuaries of Australia published a paper entitled “Thinking about life insurance through a genetic lens” in May 2017, in which new business claim costs and lapse rates were modeled for critical illness. Depending on the percent of the population undergoing genetic testing and variation in disease incidence based on polygenic risk scores, claim costs were projected to increase by approximately 1.8%, and in-force lapse rates could average 0.5%.41
In October 2018, the Reinsurance Section of the Society of Actuaries (SOA) (U.S.) released new research exploring genetic testing and U.S. life insurance mortality.42 The model and assumptions were adapted from the previous research by the Canadian Institute of Actuaries. Two scenarios were modeled:
- Only the applicant knows the results of genetic testing, but both the applicant and insurer know the family history
- Only the applicant knows the results of both genetic testing and family history
In the first scenario, the SOA concluded future increases in expected new business claim costs would range from 4% to 8%. In in-force blocks, claim costs could rise by as much as 3%. In the second scenario, new business claim costs could rise by 5% to 10%, with in-force claim costs increasing by 4%. The SOA made many assumptions, as did the Canadian study, in its analysis. To that end, the SOA has provided a modeling tool which individual insurers can use to vary the assumptions at their discretion in order to produce an individualized assessment.
With regard to health and medical reimbursement cover, genetic testing can lead to increased earlier identification of disease risk but may also result in higher overall health care costs, as asymptomatic people will be more likely to use the information to seek specialized medical counsel and screening and to access potential new treatments due to their genetic profiles. Conversely, it is also possible that using preventative therapies guided by genetic tests could decrease the expense of healthcare costs associated with end-stage disease in the future.
Genetic testing can also lead to improved disease prognostication in clinical practice and better risk stratification for insurers underwriting those who have already developed a disease with a genetic component. It can also mitigate drug side effects and optimize medical therapies in cases where medicines and therapies have a genetic component. Finally, there is hope that individuals, once informed about their genetic propensities, may be motivated to change lifestyle behaviors such as smoking, poor exercise habits, and overeating, and perhaps even to enter multifaceted wellness programs. The literature, however, regarding impact on behaviors and outcomes remains mixed on this. It is conceivable that ultimately, more widespread use of genetics in clinical medicine and as part of insurance products could lead to improved morbidity and mortality experience, which would have favorable implications in terms of some of the insurance pricing concerns.
Use of Genetic Testing in Products and Benefits
Insurers should be careful when promoting the use of genetic testing in insurance products and benefits. Genetic test results are frequently complicated, and clinicians may not yet have the experience to interpret genetic test results and counsel patients.
In a recent survey of primary care physicians in New York State, in spite of completing formal training, only 14% were comfortable interpreting genetic test results.43 In another report on more than 2,000 U.S. women newly diagnosed with breast cancer, half of those who undergo bilateral prophylactic mastectomy after genetic testing don’t actually have mutations known to confer increased risk of additional cancers, highlighting a limited understanding among physicians and patients of the meaning of genetic test results.44 The same study also revealed that doctors who had treated fewer than 21 patients with breast cancer were less confident in discussing test results with patients, more likely to order the test without referral to a genetic counselor, and less likely to delay surgery to get genetic test results first.45
All of this research emphasizes the urgent need for genetics experts, particularly genetic counselors, as well as for educating treating physicians about the appropriate use of genetic testing and interpretation of test results. Compounding this need, however, is a shortage of genetic counselors. According to the Bureau of Labor Statistics (U.S.), employment of genetic counselors is projected to grow 29% from 2016 to 2026, much faster than the average for all occupations. Ongoing technological innovations, including improvements in lab tests and developments in genomics, are giving counselors opportunities to conduct more types of analyses.46
Insurers also have brand and reputational risk to consider. For an insurer, offering genetic testing to a policyowner could result in negative reputational impact and potential legal challenges if the insurer is perceived to be misusing test results or if insurers select genetic testing services that either cannot deliver or that produce inaccurate results (analytical validity). It is important to recall the clinical utility of much of this new genetic information is still yet to be proven in controlled studies. Additionally, insurers could be providing the consumer with genetic information that could then be used to anti-select.
Insurers must also adhere to strict privacy, confidentiality, and data security policies with regard to genetic test results, or risk negative perceptions by the public and regulators. Safeguards for medical information have been robust and longstanding and any discussion of greater rigor around these should be welcomed, but that rigor should be applied equally regardless of whether the medical information is genetic or non-genetic in nature. The answer is not to create a two-tiered system of privacy or data security where one type of medical information is treated differently from another.
There are many possibilities for insurance products to incorporate genetic testing as part of their designs. Some insurance companies in Asia are already offering the option of post-policy issue pharmacogenomic testing to their insured populations. Genetic testing could also be incorporated into a product structure for use at time of claim to improve clinical case management, avoid drug side effects, and reduce case fatality numbers.
If insurance products are developed that offer genetic testing in some manner, it will be important that the insured’s privacy is maintained as to their results. The insured also needs to be reassured that any genetic test results found post-policy issue will not affect their current in-force insurance policy. Also, the insurance industry needs to work with the clinical community to make sure that any genetic testing services offered to the insured have clinical utility, giving a favorable material impact on the health and well-being of the insured. Thus, there should be an emphasis on a correct-to-market approach over a first-to-market approach.
Use of Genetic Testing in Underwriting and Claims
It has been argued that genetic information is somehow intrinsically unique or different from traditional medical information. This concept is termed “genetic exceptionalism.” In general, the insurance industry has maintained that genetic tests should be viewed in the same fashion as any other confidential medical information obtained with consent from applicants in their declarations or from their attending physicians at the time of underwriting. Thus, underwriters might be permitted to debit or credit a case based on disclosed genetic test results as long as it is not prohibited in their respective market and if actuarially and medically justified.
On the other hand, a ban on the use of genetic test results in insurance could affect the industry’s ability to adjudicate claims, as genetic tests are now becoming an integral part of clinical diagnostics.
Conclusions
Genomics, genetic testing, and precision medicine will play a rapidly increasing role in patient care and disease prognostication and ultimately lead to improvements in morbidity and mortality.
RGA believes genetic testing should not be required for underwriting or claim adjudication.
RGA supports the concept of symmetry of information between the insurance applicant and the insurance company to benefit both parties.
It is too early to quantify the risk of anti-selection or the impact on population morbidity, mortality, and lapse experience with any degree of certainty. The insurance industry needs to continue to support and promote the development of research to gain a better understanding of the impact.
Access to genetic testing can improve patient care and could be incorporated into insurance products for policyholder benefit.