The successful sequencing of the human genome in 2003 opened the door to a broad range of scientific and commercial opportunities and challenges.
The expanding ability to acquire health information from genetic tests and apply it to screening, prevention, diagnostics, and therapeutics has already fostered enormous changes in clinical medicine, not the least of which was launching the era of precision medicine. As the pace of investigation and discovery in genetics continues to expand and accelerate, genetic and genomic testing is now playing an increasingly pivotal role in clinical medicine as well as in life and health insurance.
Information from these tests has the potential to improve population mortality and morbidity experience, but challenges continue to mount for insurers in using such information. Many countries continue to prohibit genetic test results for use in underwriting, even if the results have been shared with an insurer. In addition, as more individuals order their own genetic tests, a growing asymmetry of information continues to emerge. This may create added challenges and could signal a significant shift in the information balance between proposed insured and insurer.
Genetic testing’s impact on the insurance industry is and will continue to be an important topic of actuarial research for some time as insurers address its effect on pricing and valuation. Insurance product development opportunities are also emerging that have the potential to capitalize upon the genetics revolution and benefit both the consumer and the insurance industry.
The ultimate objective would be for insurers to partner with insureds and other stakeholders to leverage genetic advances for mutual benefit and to promote longevity and improve quality of life. RGA is strongly optimistic about the potential for genetic and genomic medicine to drive morbidity and mortality improvements and increase healthy life expectancy. Join RGA as we explore this topic in depth below:
Background: Genetics and Genetic Testing
All human genetic information is contained within the 23 chromosomes found in nearly every cell of the body The DNA in chromosomes is arranged in a very specific linear sequence of three billion chemical base pairs, which are organized in a double helix configuration Within those base pairs are the approximately 23,000 protein-coding genes, also known as the exome (See Figure 1.)
The exome makes up 2% of human DNA. The remaining 98% is noncoding DNA, which regulates gene expression and may also have other, as yet unknown, functions.
The sum of all the DNA in a chromosome, both the coding and non- coding genes, is called the genome.
Genetics is the term that refers to the study of genes and their roles in inheritance Genomics describes the broader study of the genome, including interactions of genes with one another and with the environment.2
Genomic and genetic tests, which analyze how genes contribute to health and disease, are playing a sizable and growing role in the development of gene-based treatments. Genome-wide association studies (GWAS) use microarray (gene chip) technology to examine genome-wide sets of genetic variants and produce genotypes. The single base pair genetic variants known as single nucleotide polymorphisms (SNPs), for example, may associate with factors such as higher or lower predisposition to develop a disease or propensity to experience side effects from certain drugs (see Figure 2). From these studies, polygenic risk scores (see page 8), which measure predispositions for certain genetic conditions, can be calculated.
Other genetic tests include whole genome sequencing (WGS), which decodes every base pair, and whole exome sequencing (WES), which only decodes the protein coding regions. Targeted gene sequencing panels, another type of test, consists of laboratory panels assembled to contain a preselected or custom-designed set of genes or gene regions with known or suspected associations with particular diseases.4 These gene panels are used with individuals who have symptoms of an illness with a genetic component, such as cancer, or a family history of such illnesses. These panels enable searches within the individual’s genome for specific variants associated with the impairments in question without the need to sequence the entire genome or exome, and can assist with diagnosis, prognostication, and treatment decisions.
For a comprehensive list of genetic terms and definitions please refer to the U.S. National Cancer Institute Dictionary of Genetics Terms.
Figure 1:
The Genetic Code
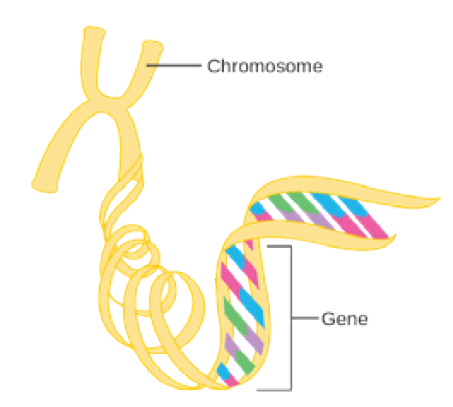
Source: Cancer Research UK / Wikimedia Commons1
Figure 2:
Source: NHS National Genetics and Genomics Education Centre3
Tests: Validity and Utility
Genetic tests, like any other medical tests, can yield false positive as well as false negative results. Whether a genetic test is applicable in a given clinical situation depends on three factors: its analytical validity, clinical validity, and clinical utility, as defined in Table 1.
Table 1: Genetic Test Criteria |
Analytical Validity | The ability of a genetic test to detect and measure the presence of a genetic variant of interest accurately and reproducibly. |
Clinical Validity | The ability of a genetic test to divide a population into two or more groups on the basis of risk or outcomes. |
Clinical Utility | The ability of a genetic test to demonstrate improvement in the outcomes (prevention, prognosis, diagnosis, treatment, or management) of an impairment. Often the term “actionable” is used when considering clinical utility. |
Source: Adapted from Merker JD, et al. J Clin Oncol.5l
In terms of analytical and clinical validity, genetic tests can reliably find variants, but as targeted gene panels do not test for all types of genetic variants, the possibility of false negative results exists.
Importantly, simply having a genetic variant does not necessarily translate into clinical manifestation of disease (i.e., the phenotype of an organism). This aspect, known as penetrance, is defined as the likelihood of a disease developing when the variant is present. Complete penetrance means 100% of individuals with a genetic variant will manifest the disease, and incomplete penetrance means less than 100% will do so. Penetrance, however, only indicates whether an individual expresses a trait associated with that gene or not. Expressivity describes the degree or extent to which the phenotype connected with the gene manifests, which can vary from person to person and depends on elements such as modifier genes, epigenetic factors, or the environment In any particular disease, expressivity could mean the difference between a very mild case in one person and a very severe case in another.6
Clinical utility refers to whether a doctor, knowing a patient has a specific genetic variation, might change how they medically manage the case. This can translate to whether a physician might motivate patients who possess potentially disease-causing genetic mutations to change behaviors or institute preventative strategies that might materially improve clinical outcomes. A 2013 study found that approximately 3% of adults carry high-penetrance, actionable, and pathogenic (or likely pathogenic) genetic variants. Knowledge of one or more these variants could enable doctors to tailor patient care to prevent or at least forestall disease expression.7 However, some critics of genetic testing argue that the presence of genetic variants might not yet be a strong enough argument for using genetic information in clinical care unless there is substantial adverse family history or clinical signs and symptoms of a particular disease.
Mutations, Polymorphisms, Variants
Genetic research has determined that all humans, no matter the geographic origin, are about 99 5% the same genetically.8 Studies of twins have shown that approximately 25% to 50% of morbidity and mortality differences from person to person are due to genetic variation.9, 10 The remaining variation in disease rates are due to environmental, behavioral, and lifestyle factors.
Two recent studies clearly demonstrate the impact of non-genetic factors on outcomes, independent of genetic determinants. One, from 2016, looked at the genetic component of cardiovascular disease (CVD) risk based on polygenic risk scores (PRS) (see page 8). It found that individuals with favorable lifestyle factors had a 46% lower relative CVD risk than those with unfavorable lifestyle factors, despite equally high genetic risk.11 Another study, conducted in 2018 and based on UK Biobank data, demonstrated that an unfavorable lifestyle profile was associated with increased risk of stroke across all genetic risk strata. These findings highlight the potential of lifestyle interventions to reduce the risk of stroke across entire populations, even for those at high genetic risk for stroke.12
In genetics, a mutation is defined as a permanent change or alteration in the nucleotide sequence of DNA. A polymorphism is defined as a variant nucleotide with a frequency above 1%. The terms “mutation” and “polymorphism” have in the past led to confusion because of incorrect assumptions about the pathogenic and benign effects of each. Both can be benign or a cause of disease. To tackle this conundrum, guidelines were issued in 2015 by American College of Medical Genetics and Genomics (ACMG) and the Association for Molecular Pathology (AMP) that recommended replacing the terms “mutation” and “polymorphism” with the term “variant.”13
As a practical example in today’s world, the COVID-19 Genomics UK Consortium’s COG-UK Project Hospital-Onset COVID-19 Infections Study (COG-UK HOCI) was initiated to evaluate the benefits of rapid COVID-19 genomic sequencing in preventing the spread of the virus in the U K ’s National Health Service (NHS) settings.79 Sequencing of viral genomes to detect closely matched sequences has been shown to better identify hospital outbreaks as compared to standard infection and prevention control measures only.
Genetic research has determined that all humans, no matter the geographic origin, are about 99.5% the same genetically.
A typical individual’s exome sequence may have approximately 40,000 variants, and a whole genome sequence approximately three million. Variants can be classified as follows:
Cell type. There are two types of cell variants: somatic and germline Somatic variants are genetic alterations that can occur in any cell of the body except germ cells These variants are generally not hereditary Germline variants are genetic alterations that occur within germ cells (egg or sperm) and can therefore be passed on to subsequent generations
Alteration types. Single nucleotide variants (SNVs) are both the most common and smallest alterations Others alteration types include indels (insertions and deletions of bases in a genome), tandem repeats, copy number variations (additions or deletions that change how many times a larger segment of a DNA sequence appears in a genome), chromosomal rearrangements (e.g., inversions and translocations), and copy-neutral loss of heterozygosity (LOH), or homozygosity.14
Clinical classification. The ACGS guidelines, which are published annually, provide a five-tier framework to describe the quantity and quality of evidence needed clinically to classify a variant (see Table 2).15 The vast majority of currently identified genetic variants fall into the category of “variant of unknown significance” (VUS). As more is learned about VUSs, many will likely be reclassified, but no clinical decisions should be made at this time on the basis of a VUS Indeed, a 2018 study by Mersch, et. al.16 found that of the 1 45 million people who received genetic testing for hereditary cancer risk between 2006 and 2016, only 7 7% of the incidentally found unique VUSs were reclassified. Of these, 8 7% were upgraded to pathogenic and likely pathogenic and 91 2% were downgraded to benign and likely benign.
Table 2: Types of Genetic Variants |
Term | Probability Variant is Pathogenic |
Pathogenic | Very high likelihood the variant is the cause of the disorder; >99% certainty that the variant is pathogenic |
Likely pathogenic | High likelihood the variant is the cause of the disorder; >90% certainty that the variant is pathogenic |
Variant of unknown significance (VUS) | Further testing or investigations could be undertaken to reclassify the variant |
Likely benign | These variants are not relevant and may be confusing if included in a report; >90% certainty that the variant is benign |
Benign | These variants are not relevant and may be confusing if included in a report; >99 9% certainty that the variant is benign |
Source: ACGS Best Practice Guidelines for Variant Classification 201915
In 2013 and again in 2017,17 the ACMG recommended that all labs performing whole exome and whole genome sequencing tests include reports of secondary findings (SF) (earlier known as incidental findings) in addition to reports of any variants found that are related to the primary purpose of the testing. Version 3 0 of this now annually updated report, which was released May 2021, includes 73 genes in its SF list which are considered medically actionable and may have a high likelihood for reducing morbidity and mortality.
Market Factors and Trends
The global genetic testing market accounted for US$12.7 billion in 2019 and is expected to reach US$21.3 billion by 2027, which would reflect a compound annual growth rate (CAGR) of 10.1% over seven years (2020 to 2027).18
In 2019, North America was the leading revenue contributor to the global genetic testing market and is expected to dominate the market over the next few years. The Asia-Pacific region is also expected to see substantial revenue growth due to greater affordability of these tests, an expected surge in healthcare expenditure, and rising awareness of the benefits of early screening for genetic disorders. In Europe, advanced healthcare infrastructure, availability of leading market participants, and advanced technology integrated with genetic testing will foster further market expansion.19
DNA sequencing looks for genes known to increase the risk of developing certain health conditions. As DNA sequencing costs continue to drop, the number of types of clinical genetic tests available has grown substantially. As of August 2017 (latest figures available), approximately 75,000 genetic tests were on the market, and a 2018 study found that on average 10 more were being added each day.20
These tests are also being used for more purposes: a genetic testing claims database found that in 2018, prenatal genetic tests accounted for 33% to 43% of spending on genetic tests, and hereditary cancer tests accounted for about 30%. 20 In 2020, however, the most utilized genetic tests were diagnostic, followed by prenatal and newborn testing.18
The cost of genetic tests has dropped markedly as well. Today they can range from less than US$100 to US$4,500, depending on the testing methodology and completeness of the assay. The cost of a whole genome sequencing, for example, is now approximately US$60021. Overall, economic activity generated by developments in genetics and genomics exceeded $108 billion in 2019 and ultimately supports more than $265 billion across the U S economy.82
Public access to genetic testing has also been increasing rapidly. Today these tests are available not just through clinicians, but also from employee health programs and directly to consumers via direct-to-consumer (DTC) genetic testing kits. The growth of DTC testing has been significant, with more than 14 million tests performed in 2018 alone. Indeed, by the beginning of 2019 more than 26 million people had contributed their DNA to four leading commercial ancestry and health databases.23
Although DTC testing is different from genetic health risk (GHR) tests, which are generally ordered by doctors, some blurring of the lines has begun to emerge. For example, on March 6, 2018, the U S Food and Drug Administration (FDA) granted the first marketing authorization for DTC tests by 23andMe of three BRCA1 or BRCA2 mutations. Permission was granted based on its analytical validity (i.e., the accuracy and reproducibility of the test). However, in its permission statement, the FDA noted that “most BRCA mutations that increase an individual’s [breast cancer] risk are not detected by this test.”24 Therefore, concern is rising that a false sense of security might be conferred by a negative DTC test.
Finally, in light of the COVID-19 pandemic, telegenetics – provision of post-test remote genetic counseling services – is increasingly becoming an effective method of clinical practice. A systematic review by Brown, et. al. (2021) showed that across most outcomes measured, telegenetics had equivalent outcomes to in-person counseling.22
Applications of Genetic Testing
Polygenic Risk Scores
Geneticists have observed that certain SNPs and SNP patterns occur more frequently in people with a particular disease or complex trait. As GWAS sample sizes have increased, more SNPs associated with disease have been discovered, which can then be used to predict an individual’s morbidity risk.
A polygenic risk score (PRS) is a metric that condenses information from tens, hundreds, thousands, or even millions (“poly”) of a person’s genetic variants (“genic”) into a score that measures their genetic predisposition for a particular disorder. These scores are often expressed as risk percentiles. An individual with a PRS in the 99th percentile for diabetes, for example, would be considered to be at high genetic risk for the disease. However, for someone with a PRS closer to the population mean (50th percentile), the score would offer no additional risk information. That person’s predicted risk, based on his or her genetics, will be similar to the population’s average risk.
Researchers have now developed PRSs for many common diseases and the scores have already shown their efficacy in risk prediction. For example, a 2016 study on genetic risk for coronary artery disease found that an individual with a PRS in the highest 5% of such scores had a threefold increased risk of experiencing the disease compared to those with lower PRSs.11 In addition, a 2015 study on genetic risk and breast cancer found that women whose PRSs were in the top 20% had a 17.2% lifetime risk of breast cancer, compared to a 5.3% lifetime risk for women whose scores were in the lowest quintile.25
Since 2016, RGA, in collaboration with King’s College London, has been conducting research utilizing UK Biobank data aimed at gaining a better understanding the value of the PRS to predict disease and death. A key focus of this research is to examine whether these scores provide additional risk information not captured by routinely collected clinical and biomarker data.
Findings have demonstrated that PRSs could make a significant contribution to risk prediction for incidence of death from breast cancer and coronary artery disease, above and beyond typical underwriting risk factors. This raises the possibility of adverse selection where genetic information about common disorders is available for insurance purchasers but not underwriters. These results have been presented at numerous actuarial conferences around the world and are discussed in detail in a series of webcasts.
Further study continues to be imperative to understand how PRSs and other advances in genomic medicine could cause adverse selection if consumers use this information to alter their insurance purchasing behavior. Nonetheless, the hugely positive impact genomic medicine is likely to have on reducing morbidity and mortality may help to mitigate this risk. For example, on April 29, 2021, the U.K.’s National Health Service launched a polygenic risk score trial for heart disease. The aim of the trial is to improve risk prediction for cardiovascular disease so that therapies such as statins as well as lifestyle changes can be targeted to the individuals who would benefit most.26
Epigenetic Testing
Epigenetics is a rapidly growing field of genetic science that studies how gene expression can be affected by changes unrelated to the underlying DNA sequence. The word “epigenetics” is derived from Greek and literally means “over and above the genome.”27 It refers to how exposure to external factors such as environmental pollution, tobacco, alcohol, diet, and lifestyle can induce chemical modifications that change how a human genetic code is expressed.
The changes are characterized by DNA methylation, histone modifications, or higher-order structural modulation of chromatin (the components of chromosomes). While heritable, many epigenetic changes can be reversed.
DNA methylation is an epigenetic process which occurs via the addition of small chemical tags (called methyl [CH3] groups) to DNA, switching genes on or off. Epigenome-wide association studies (EWAS) provide supporting evidence that external factors leave traceable methylation patterns, which can be identified using a DNA methylation test. There are currently more than a dozen DNA methylation biomarkers registered with the FDA.28 Tests can be carried out using non-invasive saliva samples or blood samples, and results can help indicate a person’s state of health and wellness.
According to the National Genomic Data Centers, the top ten areas in epigenetic studies are smoking, aging, BMI, Type 2 diabetes, maternal smoking, alcohol consumption, waist circumference, breast cancer, gestational diabetes, and depression.77
Initial epigenetic tests have focused on tobacco use and alcohol consumption DNA methylation can provide information on lifetime usage of tobacco and alcohol, and can also distinguish between current and past as well as light and heavy users. Epigenetic tests can also help determine a person’s biological age, known as the “epigenetic clock,” which may be altered by diet, exercise, and environmental factors.29 The difference between epigenetic age and chronoIogical age is referred to as “acceleration” or “deceleration.”77
Links between epigenetic age and mortality have been established in numerous populations globally. In fact, a randomized-controlled study published in April 2021 demonstrated that specific diet and lifestyle interventions may reverse epigenetic indicators of aging in healthy adult males.30
Several DNA methylation tests are now available. A DNA methylation blood test, EpiSign, was launched in 2019 by Greenwood Diagnostic Labs in conjunction with the London Health Sciences Centre. It was developed to identify unique epigenetic signatures and methylation abnormalities for more than 40 recognized genetic conditions, including Fragile X syndrome and Prader-Willi syndrome. Two DNA methylation tests from Behavioral Diagnostics LLC, Smoke Signature and Alcohol Signature, quantify smoking and alcohol consumption. The cost of these tests can vary, with EpiSign costing US$1,500, Smoke Signature US$49, and Alcohol Signature US$99, but the benefits from improved underwriting risk assessment leading to reduced insurance claims costs could be much larger.31, 32
Saliva testing, commonly used in DNA methylation testing, may also be a useful tool to add to a routine underwriting assessment Saliva can be collected by a doctor or paramedic, or even self-collected by the applicant and sent directly to a laboratory for epigenetic screening.33 As laws regarding the use of genetic test results do not yet clearly prohibit the use of epigenetic information, results of these tests could speed the underwriting process, allow for more accurate risk assessment, and benefit the life insurance applicant by providing useful health and wellness information.77
Epigenetics is a rapidly growing field of genetic science that studies how gene expression can be affected by changes unrelated to the underlying DNA sequence.
Molecularly Targeted Therapy for Cancer
Genetic and genomic testing has revolutionized the field of oncology. The tests now available to assess cancer cells are providing an increased understanding of the underlying key pathways and molecules involved in tumor growth and progression. From these tests, new molecularly targeted therapies are rapidly being developed.
Molecularly targeted therapies use an agent (such as a drug) or a combination of agents to act specifically on a defined target or pathway involved in tumor growth and spread. The improved specificity provided by these therapies should theoretically improve the therapeutic response to treatment by selectively destroying tumor cells while at the same time causing little to no harm to normal cells.
Genetic and genomic testing has revolutionized the field of oncology.
The number of targeted molecular therapies for cancer now available, as well as cancers treatable with these therapies, continues to grow. Some of the more common targeted therapies in use and the cancers they may be used to treat are:
- Signal transduction inhibitors. These agents block certain signals that pass from one molecule to another inside a cell. As a result of signal alteration, cell growth, differentiation, and survival may be affected.34 Imatinib mesylate, the first tyrosine kinase inhibitor approved for cancer therapy, is a type of signal transduction inhibitor and is used to treat certain chronic leukemias.
Proteasome inhibitors. A key cellular process in the human body is the balance between protein synthesis and degradation. Proteasomes are at the center of the protein degradation regulatory network and are critical for cell survival,35 as the proteasome system is responsible for the degradation of 80% of human cellular proteins. An example of this class of medications is bortezomib, which is used in the treatment of multiple myeloma and mantle cell lymphoma. Its action is primarily proteasome inhibition, but the mechanisms that eventually lead to selective cancer cell death are not entirely clear at this point.
Angiogenesis inhibitors. This class of medications blocks the formation of new blood vessels. Growing tumors activate the formation of blood vessels in order to meet the tumor’s demand for oxygen and nutrients. Angiogenesis inhibitors such as bevacizumab limit tumor blood supply and are used to treat: certain brain tumors; cancers of the kidney; neuroendocrine tumors of the pancreas, gastrointestinal tract, and lung; and certain breast cancers.78
Monoclonal antibodies. These agents can impact cancers in several ways. When used as immunotherapy they stimulate a person’s own immune system to target cancer cells. They can also be used in targeted cancer therapy, either alone or as a vector, to deliver drugs into or onto a targeted cancer cell to kill it. Some examples of targeted monoclonal antibodies are trastuzumab, which is used for some breast cancers, and alemtuzumab, which is an effective treatment for certain leukemias.
Unfortunately, these novel targeted cancer medications are expensive and, in many cases, only extend life expectancy marginally. A study conducted in 2018 examined the percentage of U S patients with advanced or metastatic cancer who benefited from genome-driven oncology between 2006 and 2018. Utilization of genome-informed cancer therapy rose from 10.5% to 15.4% and persons benefiting from this therapy increased from 1.3% to 6.6%. The median overall response rate for all genome-informed drugs through January 2018 was 54%, with a median life expectancy increase of 29.5 months. The study authors concluded that these novel drugs have helped only a minority of patients with advanced cancer.37
Liquid Biopsies
Considerable focus and research have been directed in recent years to the development of liquid biopsies. These tests, which look for biological and genetic markers of cancers in blood or other bodily fluids, have been studied for screening and therapeutic purposes, including the surveillance of recurrent or metastatic disease. There is a high expectation that these tests may have significant and beneficial impacts on mortality and morbidity outcomes of cancers in the coming years.
The National Health Service in England announced on November 27, 2020 that it was piloting Galleri, a potentially revolutionary blood test purported to detect more than 50 cancers.38 This blood test, developed by GRAIL, Inc , can differentially detect cancers in various stages of development. It is being piloted in two groups of patients totaling 165,000 individuals. The first group consists of 140,000 individuals ages 50 to 79 who were identified through their health records and who have no cancer symptoms. They will undergo blood tests annually for three years and will be referred for investigation if a test result is positive. The second group will include 25,000 people with potential cancer symptoms. These patients will be offered the Galleri blood test to speed their diagnoses after referral to a hospital via normal channels. Results of the pilot are expected in 2023. If successful, the test will be rolled out to one million individuals in 2024 and 2025. This test could help meet the NHS’s Long Term Plan goal of increasing the proportion of cancers caught early, which could be key to reducing cancer mortality. (Patients whose condition is diagnosed at stage 1 typically have between five and 10 times the chance of surviving compared with those found at stage 4.)80
Demonstrating the potential benefit of liquid biopsies, the interim results of the PATHFINDER study, a multi-center study of 6,662 participants in the U.S. age 50 and older, showed that more than half of new cancers were detected at early stages (I-III) using a multi- cancer early detection test.
There is some concern that liquid biopsies could impact cancer incidence rates and cancer diagnostic definitions, and thus challenge actuarial pricing assumptions for living benefits products. Insurers should remain vigilant and anticipate the possibility of potentially needing to address pricing issues in the future.
For a white paper providing a detailed review of this testing and RGA’s opinion, please click here. [Note: this paper is scheduled for updating in late 2021.]
Pharmacogenomics (PGx)
Personalized medicine, now known as precision medicine, is the practice of clinical decision-making such that the decisions made maximize the outcomes for the individual patient. Pharmacogenomics is an area within precision medicine which studies how the genome affects the metabolization of drugs, an individual’s response to different drugs, and how treatment can be adapted to an individual’s genetic makeup. Pharmacogenetics is somewhat different in scope: it is the study of the specific genetic variations that cause different responses to drugs, depending on the person. While the two areas overlap, pharmacogenomics takes a wider look at the overall genetic picture, rather than focusing on how single genes affect the action of pharmaceuticals.
Genetic variations that exist from person to person can play a fundamental role in the treatment outcome of diseases such as cancer, human immunodeficiency virus (HIV), heart disease, and hypertension. It has been estimated that 97% of individuals have high-risk pharmacological variants in their genome, which can affect drug absorption, distribution, metabolism, and excretion.39 Variants can be influenced by heritability as well as epigenetic changes due to exercise, diet, smoking, and air pollution.
A number of organizations provide information on genetic variations that affect responses to medications. The Clinical Pharmacogenetics Implementation Consortium (CPIC), for one, uses PharmGKB Clinical Annotation Levels of Evidence, the scoring system developed by Pharmacogenomics Knowledgebase, to assign specific levels (A, B, C, or D) to drugs, based on supporting pharmacogenomic evidence.40,41 PharmGKB is a U.S. resource funded by the National Institutes of Health (NIH) that provides information about how human genetic variation affects response to medications A-level drugs have the highest level of actionability and D-level drugs the lowest. There are currently 442 gene-drug combinations listed on the site.
The FDA publishes a list of approved drugs with pharmacogenomic labeling, currently totaling 178, which includes information on indications for use, dosage recommendations, and warnings. The annual proportion of new FDA drug approvals with pharmacogenomic labeling has increased by nearly threefold, from three in 2000 to 11 through July 2020.42
The benefits of pharmacogenomic testing for a patient include reduced drug toxicity and better treatment efficacy, which ultimately can lead to lower morbidity and mortality. Another important consideration is cost, as prices charged for testing differ across laboratories and by country and can range from US$100-$300 to as much as US$2,000. A once-in-a-lifetime test for the polymorphic genes, which affect commonly prescribed drugs may be cheaper than the current clinical practice of carrying out regular blood tests to measure drug concentrations over a person’s lifetime. A recent study examining cost savings using pharmacogenetic testing for depression, for example, reported a savings of US$3,962 per patient per year, based on a genetic test cost of US$2,000.43
The life insurance industry could benefit from exploring opportunities to innovate by applying pharmacogenomics in product development. Pharmacogenomic benefits could be used judiciously to promote better disease control, avoid adverse drug reactions, and improve efficacy and survival rates. Of course, insurers must keep in mind the concepts of clinical utility and clinical validity (see page 5).
Other Innovations in Genetics
Gene Editing
Scientists have been searching for ways to edit genomes since the 1960s. The discovery of CRISPR-Cas9 (Clustered Regularly Interspaced Short Palindromic Repeats-[CRISPR] associated protein 9) technology in 2012 is bringing science closer to this capability.
The life insurance industry could benefit from exploring opportunities to innovate by applying pharmacogenomics to product development.
Genome editing is a group of technologies enabling scientists to modify an organism’s DNA. These technologies enable genetic material to be added, removed, or altered at particular locations in a genome. Several biologic systems are being used but CRISPR-Cas9, which is regarded by many scientists as a molecular scalpel, has generated substantial excitement because it is faster, cheaper, more accurate, and more efficient than other genome editing methods.
This innovative technology is of great interest in the prevention and treatment of human diseases. Most research is being conducted using cellular and animal models but scientists are currently working to determine whether this approach is safe and effective for humans.44 To date, CRISPR-related technologies have been shown to improve symptoms of Fragile X syndrome in mice and cure dogs of Duchenne muscular dystrophy (a rare muscle-wasting disease that in humans mostly affects boys).45
A small number of patients, mostly participants in research studies, have already been treated with this technique. In one case, CRISPR technology was used in 2019 to treat sickle cell disease in a patient. Now, two years after her blood cells were re-engineered to correct the sickle gene, healthy hemoglobin is populating her bone marrow.45
Another potentially revolutionary utilization of CRISPR technology is for a rare type of hereditary blindness. In March 2020, CRISPR technology was used on an adult human subject to treat the disease by editing the CEP290 gene, which was causing the blindness. People with this condition worldwide are awaiting preliminary results, which are due out later this year.45
Even though most CRISPR-Cas9 research is focused on single-gene disorders such as cystic fibrosis, hemophilia, and sickle cell disease, this technology also holds out hope for the diagnosis, treatment, and prevention of more complex diseases such as cancer, heart disease, mental illness, and human immunodeficiency virus (HIV).80 Early clinical trials using CRISPR-edited cells for cancer therapy began in 2018.46
In June 2021, a published report demonstrated in vivo use of CRISPR-Cas9 gene editing in patients with hereditary transthyretin amyloidosis in order to reduce the concentration of misfolded transthyretin protein in serum.47 This study, which is still in progress, revealed only mild adverse events and led to decreases of the abnormal protein. It is also the first to show that the technique can be safe and effective via direct infusion into the bloodstream.48
Genome editing technology also brings up a number of ethical challenges, as these tools have the capacity to alter human genomes. Even though most changes introduced with genome editing are limited to somatic cells, genome manipulation could be used to modify germline cells and may thus be heritable.
Based on issues about ethics and safety, and in the wake of the Chinese scientist’s edit of the CCR gene in human twins in 2018, germline cell and embryo genome editing are currently illegal in many countries.49 In 2019, the World Health Organization (WHO) established a global expert panel to examine the scientific, ethical, social, and legal challenges associated with human genome editing.50 In July 2021, WHO issued a statement in support of establishing an international governance structures for human genome editing studies, the formation of a global registry to track editing studies, the creation of a mechanism for whistleblowers to report unethical work, and in the encouragement of the equitable development of the technology.51
Currently, the scientific community is facing ethical dilemmas in light of both the ample positive potential gene editing is offering and the possibility that such edits may elicit future unintended consequences (i.e., off-target mutations). Concerns include disruption of oncogenes, tumor suppressor genes, and/or DNA repair genes, resulting in significant cellular toxicity and/or development of cancer.46 Fortunately, emerging research is likely to significantly reduce such off-target mutations.54
This innovative technology offers the promise that hereditary diseases could be more precisely treated. The next few years will show us whether it can ever be translated into safe and ethical human clinical trials.
Molecular Techniques to Treat Genetic Disorders
Two molecular techniques in addition to gene editing are currently available for possible use in humans to alter the sequence or expression of genes. They are gene therapy and gene silencing.
In gene therapy, one or more genes are inserted into a person’s cells to correct for the presence of an inherited disease-causing mutation. The resulting new, normally functioning gene is referred to as a transgene.
Gene therapy is unique in medicine as its goal is to treat the cause of a disease rather than its symptoms. Its use in clinical therapy is currently evolving and holds great promise to revolutionize the management of human disease.52 A limiting factor to date, however, is finding an efficient way (e.g., by a vector) to deliver its constructs to a person’s cells.
There have been significant developments in this field over the last five years, with several new therapies approved.53 The first-ever approved antisense oligonucleotide, nusinersen, is designed to treat spinal muscular atrophy, and the oligonucleotides eteplirsen and golodirsen are both approved for the treatment of Duchenne muscular dystrophy. Various CAR T-cell therapies, including tisagenlecleucel for the treatment of relapsed/refractory B-cell acute lymphoblastic leukemia,57 axicabtagene ciloleucel for the treatment of relapsed/refractory diffuse large B-cell lymphoma,58 and brexucabtagene autoleucel for relapsed/refractory mantle cell lymphoma, have also all been approved and are in clinical use.
In vivo gene therapies for inherited disorders include voretigene neparvovec, the first to treat Leber’s congenital amaurosis 2, a specific form of retinitis pigmentosa.56 Also of note is onasemnogne abeparvovec-xioi for spinal muscular atrophy (SMA) in children under age two. Prior to the development of this therapy, mean survival of treatment-naïve children with SMA type 1 was found to be six to eight months.59 In May 2021, an ongoing long-term follow-up safety study of 13 treated infants with symptomatic SMA type 1 found that a favorable safety profile was observed for up to 6 2 years after dosing. In addition, the therapeutic dose maintained a durable response in patients up to 5 6 years after dosing, with all patients alive and without the need for permanent ventilation. The authors noted that this would appear to be the longest follow-up of gene therapy published to date.60
The costs of these medications, however, are substantial: a one-time treatment of voretigene neparvovec is US$425,000 per eye; a one-time infusion of tisagenlecleucel is US$475,000, and onasemnogene abeparvovec-xioi, the world’s most expensive drug, has a one-time cost of US$2.1 million.
Other conditions that appear treatable via gene therapy include retinal defects,55 clotting factor deficiencies seen in hemophilia, cystic fibrosis, and certain hemoglobinopathies (inherited single-gene disorders) such as sickle cell disease, thalassemia, and certain cancers.
Gene silencing does not add or change the genetic information in a cell, unlike gene therapy or editing Instead, via molecular techniques, it works to decrease the expression of one or more genes Gene silencing is carried out via RNA interference (RNAi) technology, which consists of the use of different types of RNA, including silencing RNA (siRNAs), short hairpin RNA (shRNA), and microRNA (miRNA). Clinical use of gene silencing has been limited by the ability to achieve successful systemic delivery of these silencing RNAs to cells. The use of lipid-based nanoparticles (LNPs) that protect RNA have shown significant promise as a non-viral vector to deliver silencing RNAs, as evidenced by the recent development of messenger RNA (mRNA)-based vaccines for COVID-19.61 A few clinical applications of gene silencing are currently being utilized or investigated for hemophilia, hemoglobinopathies, Huntington’s disease, amyotrophic lateral sclerosis (ALS), and amyloidosis.
Undoubtedly, the clinical armamentarium of gene therapy, gene editing, and gene silencing will continue to grow in the future, with high expectations of reducing morbidity and mortality. However, costs, patient access, and resource allocations may present significant barriers.
Risks and Benefits for Insurers
Genetic testing is a highly charged and controversial topic – not just for the public, but also for lawmakers, regulators, and the insurance industry. There has been frequent commentary for years about the public’s concern that their genetic information might impact their insurability, which could reduce their willingness to participate in genetic research studies – something insurers need to bear in mind. For insurers, although there may be certain benefits in having access to applicant and policyholder genetic tests, there are also several possible risks.
Given the growing and pervasive importance of genetics in so many areas of science, medicine, technology, and business (including insurance), it is imperative that insurers develop an enterprise-wide genetics strategy. The purpose of this strategy would be to provide leaders and workforce members with the essential vision, guidance, and tools to achieve, consistently and cohesively, specified business objectives as they relate to genetics. RGA has formed its own Genetics Strategy Group, which comprises multiple disciplines across the enterprise, to provide subject matter expertise, lead research, and integrate genetics into the business of insurance.
Given the growing and pervasive importance of genetics in so many areas of science, medicine, technology, and business (including insurance), it is imperative that insurers develop an enterprise-wide genetics strategy.
Regulations and Risk of Anti-Selection
Currently, no insurance market anywhere in the world requires genetic tests to be ordered at the time of life or health insurance underwriting. Laws, however, vary by country and product as to whether clinically obtained and disclosed genetic test results can be used during underwriting, and these laws are constantly evolving. Most countries follow the general principle that applicants must declare what they know about all aspects of their health in order to keep the insurance contract equitable.
While most genetics regulations and laws are intended to protect consumers from perceived unfair discrimination, they can also potentially expose insurers to the enhanced risk of anti-selection or may influence consumer purchasing behavior. However, if population morbidity and mortality were to improve significantly as a result of increased genetic testing, consumers and insurers could benefit.
The application and interpretation of various laws, regulations, and moratoria around testing can be quite difficult and challenging for insurers. As types of genetic and genomic tests become more complex, they may not fit easily into the provisions or the language of written guidelines and agreements. As an example, crafting language that differentiates between a predictive and a diagnostic genetic test, which some laws and regulations refer to, can be anything but simple.
In the U.K., the Association of British Insurers (ABI), together with the British government, updated its Code on Genetic Testing and Insurance (previously called the Concordat and Moratorium on Genetics and Insurance) in October 2018. This update, still in force, is the sixth iteration of a long-standing agreement. While the name has changed, the overall directive has not: the only real change is that the agreement now has no expiration date and can be reviewed at any time.62
The U.K. as well as some European Union members have agreed to insurer moratoriums on the use of genetic information. Underwriters are required to ignore any genetic test results – even if disclosed – if the sum assured being applied for is below a certain threshold. For instance, the use of Huntington’s disease genetic information is allowed in the U K when underwriting life policies with face values greater than £500,000.
Other countries have imposed a complete ban on underwriting cases based on any disclosed genetic test results. In 2017, Canada enacted the Genetic Non-Discrimination Act (GNDA), which prohibits any person from requiring an individual to undergo a genetic test or disclose the results of a genetic test as a condition of providing goods or services to, entering into or continuing a contract or agreement with, or offering specific conditions in a contract or agreement with an individual. Exceptions are provided for healthcare practitioners and researchers. The law clearly applies to insurers and as a result, adjustments to underwriting practices in Canada have been implemented.63
There have also been additional discussions about the applicability of the law to family histories, but no firm decisions have been made In response to legal challenges to the Act, the Supreme Court of Canada ruled in July 2020 that the GNDA is a constitutional exercise of the country’s federal powers.63 (A panel of the Court of Appeal of Quebec had previously struck down the law on constitutional grounds.64)
In the U.S., the Genetic Information Nondiscrimination Act of 2008 (GINA), a federal law that prohibits genetic discrimination in health insurance and employment, does not apply to life, disability, and long-term care cover. Recently, however, there has been significant legislative activity at the state level to limit the use of genetic information in life underwriting. On July 1, 2020, Florida enacted a bill which prohibits life and long-term care insurers from canceling, limiting, or denying coverage or adjusting premium rates based on genetic information. The bill includes language stating it should not be construed as a vehicle to prevent a life or long-term care insurer from “accessing an individual’s medical record as part of an application exam” or from “considering a medical diagnosis included in an individual’s medical record, even if a diagnosis was made based on the results of a genetic test.”65 Other states are introducing similar legislation based on the Florida model.
Australia’s Financial Services Council, on June 21, 2019, published FSC Standard No 11: Moratorium on Genetic Tests in Life Insurance. The purpose of the Standard, which took effect July 1, 2019, is to ensure people can access a level of life insurance without being asked to disclose the result of a previously taken genetic test. This Standard is to be effective through June 30, 2024, with a review in 2022. Specifically, insurers may only ask for or use genetic test results if the total amount of cover, in Australian dollar aggregate, is more than any of the following:
- $500,000 of lump sum death cover
- $500,000 of total permanent disability cover
- $200,000 of critical illness cover
- $4,000 per month of income protection cover
Additionally, an insurer must take into account a favorable genetic test result disclosed by an applicant or evidence-based preventative treatment, each of which may reduce the possibility of developing a heritable illness.66
Singapore enacted The Moratorium on Genetic Testing and Insurance, which is an agreement between the Ministry of Health (MOH) and the Life Insurance Association (LIA) on the use of genetic test results in insurance underwriting. It has been phasing in since January 2021 and will apply to all insurers and reinsurers licensed to operate in Singapore. The moratorium distinguishes between diagnostic and predictive genetic tests, but only regulates predictive tests. The use of diagnostic genetic test results is left to the discretion of individual insurers. It also makes provision for the appointment of a nominated genetics underwriter (NGU) who will need to undergo mandatory accredited training and has defined liaison responsibilities. The moratorium is open-ended, with no expiration date. Specifications vary by product and sum assured, but only predictive testing for Huntington’s disease and BRCA 1 and 2 are currently allowed to be considered in underwriting.67
In 2020, Hong Kong issued its most recent moratorium on the Use of Genetic Test Results (“Best Practice”) with the effective date of June 1, 2020. The scope of the moratorium covers the use of genetic test results in relation to insurance activities, including but not limited to underwriting and claims assessment Its recommendations specifically address predictive and diagnostic genetic tests and allows insurers to use diagnostic tests for underwriting purposes. Depending on the product and sum assured applied for, the medical conditions an insurer may ask about and may take into account in terms of results of a predictive genetic test result include:
- Early-onset autosomal-dominant Alzheimer’s disease
- Hereditary breast and ovarian cancer syndrome
- Lynch syndrome (hereditary non-polyposis colorectal cancer)
- Autosomal-dominant polycystic kidney disease
- Huntington’s disease
- Hypertrophic cardiomyopathy
As for anti-selection, a study conducted more than 10 years ago demonstrated that there is a 5 7-fold increased tendency for policyowners to change their long-term care insurance coverage if they know they carry genes associated with increased risk for Alzheimer’s disease.68
RGA has been a consistent advocate for a level, free, and fair exchange of information between the insurer and the consumer. Such exchanges can provide good opportunities for partnership going forward, in terms of leveraging the information for shared benefit and ultimately lower prices for consumers.
Impact on Product Pricing
The definitive impact of genetic information on product pricing due to the information asymmetry that may stem from insurers’ ability (or lack thereof) to access genetic testing information is currently unknown. At this point it is known that genetic testing may improve both mortality and morbidity outcomes and influence in-force lapse rates. For example, someone receiving favorable genetic test results may be more inclined to allow a policy to lapse, whereas someone receiving concerning results may be more motivated to keep their policy in force, thus negatively impacting a pool’s overall mortality expectations. Much uncertainty exists, and more precise pricing assumptions about genetic testing impacts are needed both for consumer behavior and for mortality and morbidity outcomes.
Research conducted in Canada in 2011 found that the effect of restricting the use of genetic test results in underwriting would be minimal – about a 1% to 3% increase in premiums However, only six genetic disorders were included in that analysis.69 Since then, more research has been done to clarify the issues as testing continued to broaden its impact. A 2014 report from the Canadian Institute of Actuaries, for example, examined 13 impairments with a known genetic marker and found that banning the use of genetic test results in underwriting could increase average mortality rates by 35% for males and 60% for females.70 The same author, in a 2016 report, demonstrated that a ban on using genetic information in critical illness underwriting would result in a 26% increase in the average CI claims rate (+16% for males and +41% for females),71 and could necessitate an increase in CI premium rates.
In May 2017, The Institute of Actuaries of Australia published a paper titled “Thinking about life insurance through a genetic lens,” in which new business claim costs and lapse rates were modeled for critical illness. Depending on 0 5% percent of the population undergoing genetic testing and variation in disease incidence based on polygenic risk scores, claim costs were projected to increase by approximately 1 8% and in-force lapse rates by an average of 0 5%. Results also indicated that if genetic tests were to become more widely used, their potential impact on claim costs and write-off of acquisition costs due to lapse could be material If, for example, the proportion of the population tested were to increase to 2%, claims costs could rise by as much as 7% and lapses by 1 9%.72
Then, in October 2018, the Reinsurance Section of the Society of Actuaries (SOA) (U.S.) released research exploring genetic testing and U.S. life insurance mortality.73 The model and assumptions were adapted from previous research by the Canadian Institute of Actuaries.
Two scenarios were modeled:
- Only the applicant knows the results of genetic testing, but both applicant and insurer know the family history
- Only the applicant knows the results of both genetic testing and family history
In the first scenario, the SOA concluded future increases in expected new business claim costs could range from 4% to 8%, and in-force claim costs by 3% In the second scenario, new business claim costs could rise by 5% to 10%, and in-force claim costs by 4%. The SOA made many assumptions, as did the Canadian study, in its analysis. To that end, the SOA has developed a modeling tool, which insurers can use to vary assumptions for their companies at their discretion in order to produce an individualized assessment.
RGA has also produced its own research and analysis to assess the impact of genetic regulations on pricing. The robust methodology took into consideration market characteristics such as genetic testing rates (percentage of people who underwent genetic testing), the potential impact of non-genetic factors, and local regulatory environments. It also acknowledged that genetic marker prevalence and penetrance rates vary by geography, and adjusted the model for customer behavior, healthcare system characteristics, and the degree of primary care provider (PCP) involvement in patient care.74
Genetic testing in a healthcare setting and on medical advice is likely to play an increasingly significant role in patient care and disease prognostication, which could lead to improvements in morbidity and mortality. However, the growth of DTC genetic testing, which generally does not follow strict clinical recommendations for analysis, raises concerns about the potential for information asymmetry.74
DTC testing is giving individuals with or without signs and symptoms of disease easy access to a range of genetic tests, including comprehensive evaluations It is well-recognized that the predictive value as well as the analytical and clinical validity of genetic testing is greatly reduced if done in an asymptomatic individual or for someone without a significant family history. Nevertheless, the underlying motivations for undergoing genetic tests, how such testing is carried out, and how the information is handled within the context of insurance, have the potential to impact products, markets, and operations. Advances in genetic testing and the emergence of more accurate predictive genetic tests, especially in the context of family history unknown to the insurer, could significantly alter the relationship dynamics between life insurers and policyholders, exposing insurers to a substantial unexpected additional risk.74
A January 2021 report from Medscape in collaboration with 23andMe highlighted a rapidly emerging shift in the primary care community’s view of genetic testing.75 Compared to two years ago, primary care physicians are now twice as likely to be comfortable discussing the benefits, risks, and limitations of genetic health testing. As more people become interested in their genetic and genomic information, it is encouraging to see healthcare providers more open to patients bringing their test results to their clinicians for further counseling, interpretation, and appropriate follow-up as needed.75 This shift could equip the person being tested with knowledge of whether an identified genetic variant is related to the presence or absence of risk of a specific disease. It could also enable physicians to design tailored management plans that might delay or even prevent disease onset. As for insurers, it could provide access to valuable information – interpretation of these tests in a clinical context – which could lead to better mortality and morbidity risk classification when underwriting.74
With regard to health and medical reimbursement cover, genetic testing (much like increased screening) can lead to a rise in earlier identification of disease risk It may also, however, result in higher overall health care costs, as asymptomatic people may be more likely to use the information to seek specialized medical counsel and screening and access possible new treatments due to their genetic profiles. Conversely, using preventative therapies guided by genetic tests could also decrease future healthcare costs associated with end-stage disease.
Genetic testing may also lead to improved disease prognostication in clinical practice and better risk stratification for insurers underwriting individuals who have already developed diseases with genetic components. For treatments with a genetic component, testing could also mitigate side effects and optimize the therapies. Finally, there is hope that individuals, once informed about their genetic propensities, may be motivated to change lifestyle behaviors such as smoking, poor exercise habits, and overeating, and perhaps even to enter multifaceted wellness programs. Peer-reviewed literature regarding impact on behaviors and outcomes, however, remains mixed on this.
Genetic testing in a healthcare setting and on medical advice is likely to play an increasingly significant role in patient care and disease prognostication, which could lead to improvements in morbidity and mortality.
Whether people improve behavior through lifestyle changes and use genetic information to purchase more insurance cover are important areas of research that are being closely monitored by the RGA behavioral science team.74 It is conceivable that ultimately, more widespread use of genetic information in clinical medicine and as part of insurance products could lead to improved morbidity and mortality experience, which could have favorable implications for pricing. Overall, mortality and morbidity experience due to access to genetic information, anti-selection, and lapsation trends will take time to determine. However, these considerations have to be acknowledged and addressed, both in terms of various operational processes and market-wide impact.74
Use of Genetic Testing in Products and Benefits
Care should be taken when promoting the use of genetic testing in insurance products and benefits Genetic test results are frequently complex, and clinicians may not yet have sufficient experience to interpret these results and counsel patients (although this situation is improving).
Insurers also have brand and reputational risk to consider. For an insurer, offering genetic testing to a policyowner could result in negative reputational impact and potential legal challenges. This can happen if the insurer is perceived as misusing test results or if insurers select genetic testing services that cannot provide reliable analytical validity, either because they cannot deliver results or that they produce inaccurate results. It is also important to recall the clinical utility of much of this new genetic information is still yet to be proven in controlled studies. Additionally, insurers that make these tests available to policyholders could be providing genetic information that could then be used by the insureds to anti-select.
Insurers must adhere to strict privacy, confidentiality, and data security policies with regard to genetic test results or risk negative perceptions by the public and regulators. Safeguards for medical information use are longstanding and robust, and any discussion of greater rigor around these should be welcome. However, such increased rigor should be applied equally, whether the medical information is genetic or non-genetic in nature. The goal should be not to create a two-tiered system of privacy or data security where one type of medical information is treated differently from another.
It is clear that progress in our understanding of genetics poses challenges and opportunities. Recent product examples showcase how insurers are leveraging the opportunities. In Asia, a few insurers are now including additional benefits for gene expression profiling for breast cancer (developed by RGA) as well as providing medical insurance products with embedded pharmacogenomic testing benefits.74
Care should be taken when promoting the use of genetic testing in insurance products and benefits.
As an example, breast cancer genomic testing can be carried out to determine the risk of breast cancer recurrence and whether a patient will benefit from chemotherapy. Testing can also help clinicians make more informed decisions on the type of chemotherapy drugs to use, improving long-term prognosis for the patient. Examples of genomic tests profiling genes associated with breast cancer recurrence and adjuvant chemotherapy planning include the Breast Cancer Index, Endopredict, MammaPrint, Oncotype Dx, and Prosigna.83
At the start of the product development process, principles of analytical utility, clinical utility, and clinical validity, as well as accuracy, counseling support, and overall benefit(s), need to be considered to best serve both the insurer and applicant or policyholder. Furthermore, as genetic testing increasingly becomes part of standard medical care, it could trigger an improved alignment of existing products or benefits, such as CI definitions or continuous cancer benefits.
As the market prepares for more exposure, progress, and engagement with genetic information, the time to analyze and test the potential business impact is now. However, this process requires a robust methodology. Genetic science, as well as behavioral and market-specific insights, will all need to be examined when developing assumptions.
While these are complex considerations now, more will be known over time RGA closely follows genetics updates in fields beyond insurance and engages in information-gathering initiatives such as surveys. On June 7, 2021, RGA published findings from its global industry genetics survey, results of which can be viewed here.76
RGA also collaborates with experts, academics, and research institutes to study the potential impact of genetics on the insurance industry and engages in industry-wide discussions to be able to provide expert advice and information to clients. Based on these principles, RGA regional experts have developed a flexible and scalable model to assess the possible impact of genetic testing that can be easily adapted for different products, markets, and insurers.
If insurance products are developed that offer cover for and/or access to genetic testing in some manner, it will be important that the test-taker’s results remain private. Insureds will also need to be reassured that any genetic test results that emerge after policy issuance will not affect their in-force policy. Also, the insurance industry needs to work with the clinical community to make sure that any genetic testing services offered to insureds have clinical utility and can have a favorable material impact on an insured’s health and well-being. Thus, there should be an emphasis on a correct-to-market approach rather than a first-to-market approach.
Use of Genetic Testing in Underwriting and Claims
It has been argued that genetic information is somehow intrinsically unique or different from traditional medical information. This concept is termed “genetic exceptionalism.” In general, the insurance industry agrees that genetic tests should be viewed in the same fashion as any other confidential medical information obtained with consent from applicants, whether from declarations or attending physician’s statements, at the time of underwriting. Thus, underwriters might be permitted to debit or credit a case based on disclosed genetic test results as long as doing so is not prohibited in their market and if actuarially and medically justified In terms of governance, some markets require a dedicated underwriter to keep a record of cases that contain any genetic information.
It is incumbent on insurers as well to demonstrate both technical proficiency and research-based documentation if any genetic test result is to be used in risk assessment and claims adjudication. Similarly, insurers should consider any mitigating interventions being taken by a proposed insured in order to reduce disease risk connected to a genetic test result. Due to limited information, lack of long-term studies, and technical considerations which may be unknown to underwriters today, the risk assessment process for genetic information may be complex at first. Risk assessment outcomes could be dynamic over time as new information comes to light Insurers will also need to keep abreast of progress in the field of genetics as well as any potential impact on mortality and morbidity outcomes.
Overall, any impact on mortality and morbidity stemming from access to genetic information, whether anti-selection or lapsation trend changes, will take time to determine. However, these considerations have to be acknowledged and addressed in various operational processes and market-wide impacts need to be assessed.
Bans or limits on insurer use of genetic test results could affect the industry’s ability to assess and adjudicate claims, as genetic tests are now becoming an integral part of clinical diagnostics. However, if the genetic test is considered diagnostic or contributes to a clinical definition of disease, it will likely be admissible and will help ensure a fair assessment of the claim.
Conclusion
Rapid and ongoing advances in genomic medicine are remarkable achievements, which are having and will continue to have significant impact on clinical and insurance medicine as well as the overall insurance industry. It is only by staying up-to-date with developments, including risks and opportunities, that insurers will be able to successfully navigate this new and complex world While challenging, the benefits are clear.
Regarding genomics, it is incumbent upon insurers to understand the issues and concerns of consumers and other stakeholders and act appropriately, not only with regard to compliance with regulations, but in good faith, integrity, and fairness.
Additionally, RGA supports the following pillars:
- Equal access to medical information, including genetic information, between insurance applicant and insurer benefits both parties. Limited or no access may require, in the future, pricing increases to counterbalance the asymmetry of information.
- Mandatory genetic testing should not be an underwriting or claim requirement. However, genetic tests disclosed at time of underwriting or claim, where legally permitted, should be evaluated using strict and evidence-based principles.
- Provision of genetic testing may improve wellness, healthcare, morbidity, and mortality, and can be successfully incorporated into insurance products for policyholder benefit.
- Insurers must adhere to strict privacy, confidentiality, and data security practices with regard to genetic test results, as would also be mandated for other personal and confidential information.
The genomic revolution has arrived, and consumers and insurers alike will share in the benefits of that revolution. RGA is optimistic and enthusiastic about what is yet to come. Embrace, engage, and expect more great discoveries in the near future!
For more information, or if you have questions and comments, please contact the authors of this paper or any of the members of the RGA Genetics Strategy Group We look forward to assisting you and your company.